By Candace Grimes
Have you ever been stuck in a crowded room and noticed a rise in temperature? Imagine being in a confined space with hundreds to thousands of your closest friends and family. Does that sound hot and sticky (and slightly claustrophobic) to you? This is exactly what the daily life of a honeybee is like. Honeybee hives are congested colonies of thousands of bees that constantly produce carbon dioxide and heat. So how do they deal with this hot and sticky environment? They flap their wings and fan their way to a cooler more suitable temperature!
Dr. Jake Peters (Fig. 1), a postdoctoral fellow in the Applied Math Lab at the Harvard University John A. Paulson School of Engineering and Applied Science, observed honey bees located near the entrance to their hive flapping their wings (Video 1). But why? His presentation at SICB 2019 in Tampa, FL, discussed this behavior in detail. Dr. Peters has been studying honeybee behaviors, such as ventilation strategies and swarming patterns.
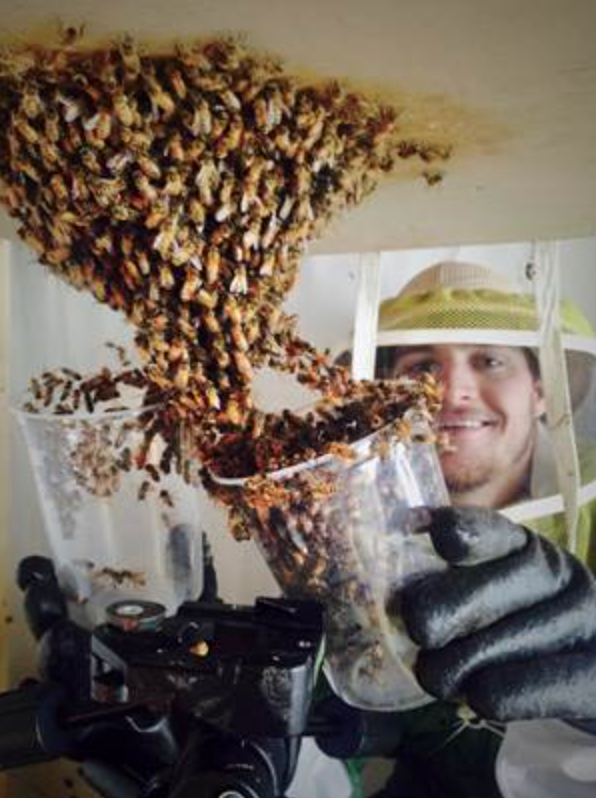
With the help of his colleagues, Drs. Orit Peleg and L Mahadevan, he noticed the bees were not evenly distributed at the entrance to the hive, but instead seemed to have “assigned seats”. These bees would place themselves in response to some cue, possibly temperature, and begin fanning their wings at the entrance of the hive. Mathematical models showed that the position and movement of the bees generated an increased airflow through the interior of the hive. Through further observation, they documented some bees have different temperature thresholds and will start fanning their wings at different times. They work together in this collective strategy to keep the hive cool and remove gaseous CO2 build up. Previous work suggested that honeybees use a tidal ventilation strategy meaning that they will “draw out” the air from within the nest by fanning then let the outside air fill the nest without expending any energy (Southwick and Moritz 1987).
Dr. Peters and his colleagues describe the two methods of air flow control shown in these honeybees as: temporal or spatial. For temporal control, the bees fan for an allotted time (depending on the temperature) and repeat when necessary. Alternatively, for spatial control, they will place themselves in an orientation to create the proper flow to remove the excess CO2 and heated air from the hive. This will allow for the typically cooler air outside the hive to flow in passively. In Fig. 2b, the high temperatures on the left side of the hive show a higher density of fanners creating the airflow shown in Fig.2a. This collective response to take care of the hive is common in other insects as well, such as ants.
Figure 2. Image from Peters et al. (2019) showing the airflow created by the bees flapping near the entrance to the hive. Caption from the article: “(a) A schematic illustrating the path of air through the hive as induced by fanning bees. Warm, stale air is drawn out of the entrance by fanning bees and relatively cool ambient air enters passively where fanning bees are absent. The camera indicates the viewing angle in (b) and (c). (b) A thermal image of the hive entrance at night when fanning bees are actively fanning. Notice inflowing air at the right of the entrance has cooled the wood and the outflowing air induced by fanning bees on the left has warmed the wood. (c) Honeybees ventilating at the entrance of a hive. Note the dense group of fanning bees at the left of the entrance and the absence of fanning bees at the right of the entrance.”
To investigate the ecophysiology of these hives, Dr. Peters (with the help of a local electrical engineer) created tiny robotic bee models to study the ventilation strategies of honeybees. Initially, one might think these tiny robot bees help cool down artificial and natural colonies, but these models are not as efficient as real honeybees. While it would be great to give bees little tiny A/C units, his models are not applicable for real hive A/C because honeybees are more efficient than the robot models. Although mini bee A/C units are unlikely, these robotic models are useful for understanding hive geometry, estimating the most efficient hive size for productivity, and whether it is more efficient for airflow to have one hive opening or two? Data might show that there is better ventilation with multiple openings. We may be able to use information from their bee robot models to redesign the hive structures currently in use to better benefit the bees and human-made hives for agricultural purposes.
Among beekeepers, the 3 most popular hive styles in most of the world are the Langstroth, Warré, and Top-Bar, each of which with their own pros and cons. Before a prospective beekeeper embarks on their journey to owning a hive, they must decide which style best suits their needs. Dr. Peters used 4 Langstroth hives for his study which are boxes with a single slit entrance at the base that can be expanded by adding boxes to the top box. While this style is not a natural structure for the bees, it does allow for better ventilation than the Top-Bar. It also allows for easier clustering during colder temperatures which the Top-Bar does not. The Warré and Top-Bar hives seem to be more aesthetically pleasing and less of a utilitarian style than the Langstroth, but they are typically more expensive, produce less honey, and/or more difficult to handle.
Figure 3. Here you can see honeybees shown at the entrance of a hive with sensors monitoring the heat and air flow near the entrance of the hive.
Figure 4. Honeybee models (aka robot bees!) that can remove the hot stale air from the hives! These models consist of a power source, fan, and electrical circuit to control when the models are turned on and off to create the proper flows.
Due to the relatively high metabolic rate of flying insects, they use morphological adaptations and behavioral modifications to adjust to increased temperature in their environment. Dr. Peters says, “Hopefully, honey bees have enough genetic diversity to survive global climate change”. Since movement requires substantial energy, this fanning behavior may increase the metabolic rate of honeybees, which will increase their energy expenditure. If their habitats are increasing in temperature, this may lead to a stressed future environment with a need for an increase in fanning behavior and increase the need for sustenance. With global climate change on the horizon and the threatened nature of bees at present, understanding these strategies for hive maintenance in these important pollinators is essential for commercially important plants.
While these bee models help researchers study ventilation strategies, the underlying research theme is how these honeybees are responding to environmental fluctuations. This leads to another aspect of Dr. Peters research which includes shaking bee swarms, which I would not recommend trying that one at home. Honeybees tend to swarm when a new queen bee is about to emerge. The existing queen will leave the present hive with about half the colony of worker bees forming a swarm in search of a new hive location, perhaps a hollow log or even the wall of a house. This swarm may take on various shapes and sizes in response to environmental conditions, such as wind turbulence. These researchers would shake these hives at different accelerations and frequencies to mimic a natural location of a typical hive, such as a tree branch, and how they respond to this wind turbulence. Dr. Peters and his colleagues recently published a paper on the mechanical adaptations of swarms to this stimulus by monitoring a hive on a shaking surface and documented how the bees responded, cluster or spread (Video 2). They found that the honeybees would spread out in response to shaking as if to stabilize the hive over a larger surface area and that the increase in magnitude of the frequency and acceleration of the shaking would increase the response (Peleg et al. 2018).
Dr. Peters has been involved in outreach with local high school teachers showing the best beekeeping strategies and hopes to continue that in the future. They aim to help beekeepers make informed choices about hive design, while also investigating the collective control strategies for hive ventilation.
Stay tuned for more research from their lab! They are currently working on a project to monitor swarm morphological changes in response to temperature fluctuations, and perhaps they can find the BEST efficient hive structure for commercial use of pollinating honey bees.
Check out these fun press releases from the lab!
https://www.seas.harvard.edu/content/how-bees-stay-cool-on-hot-summer-days
https://www.seas.harvard.edu/content/shaking-swarm
@JacobPeters10
@oritpeleg (ventilation and swarm project)
@MarySalcedo (swarm project only)
@Mahadevan_Lab
Peters JM, Peleg O, & Mahadevan L (2019) Collective ventilation in honeybee nests. J. R. Soc. Interface 16: 20180561. http://dx.doi.org/10.1098/rsif.2018.0561
Peleg O*, Peters JM*, Salcedo, MK & Mahadevan L. (2018) Collective mechanical adaptation of honeybee swarms. Nature Physics, 49, 1. http://doi.org/10.1038/s41567-018-0262-1 *equal contribution
Peters JM, Gravish N, & Combes SA (2017) Wings as impellers: honey bees co-opt flight system to induce nest ventilation and disperse pheromones. Journal of Experimental Biology, 220(12), 2203–2209. http://doi.org/10.1242/jeb.149476
Southwick EE, Moritz RF (1987) Social control of air ventilation in colonies of honey bees, Apis mellifera. Journal of insect physiology. Jan 1;33(9):623-6.