By Laura Booth, Department of Ecology, Evolution, and Environmental Biology, Columbia University
“Chipmunks jump, and
Greensnakes slither.
Rather burst than
Not be with her.”
So begins a whimsical love poem by Donald Hall that cleverly draws a connection between people and the natural world—a simple but powerful concept that drives science of all shapes and sizes.
The poem’s opener also contains the impetus for Tali Hammond’s research on chipmunk behavior. Chipmunks do, indeed, jump. They also perform many other types of behaviors—running, scratching, sleeping, and nesting (although we haven’t caught them singing and dancing in the wild yet).
As a doctoral candidate at UC Berkeley, Hammond is studying chipmunk behavior because it could help us understand effects of the most talked about aspect of the human-nature connection today: climate change.
Global Change on a Chipmunk Scale
The protagonists of Hammond’s research are two species of chipmunk—the lodgepole and alpine—both of which are native to Yosemite National Park. Over the past 100 years at Hammond’s study site in Yosemite, minimum temperatures have risen by approximately 3°C, and the lower boundary of the alpine chipmunk’s range has crept upwards by more than 600 meters. This may not sound like much of a difference to a human, but even such seemingly small changes can dramatically alter how ecosystems function. It’s an especially startling change considering that the lodgepole chipmunk, a closely related species with an overlapping range, has not demonstrated any loss in territory at all.

There are many possible approaches to answering this very question. In Hammond’s chipmunk system alone, researchers have examined genetic structure and morphology, and collaborator Rachel Walsh has examined diet changes and microhabitat use differences. By using behavioral and physiological measures, Hammond’s hope is to enrich our understanding of what mechanisms are driving these two species’ differing responses to their changing environment.
There’s only one catch.
Not only is a chipmunk small, it is a creature designed for stealth. Hammond sees chipmunks regularly while she is in the field, but only ever for a fleeting moment before they disappear up a tree or into a rock cave. This once made keeping activity budgets—the kind of detailed measurements ideal for tracking behavioral responses to temperature sensitivity, for example—nearly impossible, the stuff of ecologists’ daydreams.
No longer. Enter Hammond’s and Walsh’s collaborators: engineer Dwight Springthorpe, a graduate student in Integrative Biology at Berkeley, and Taylor Berg-Kirkpatrick, a computer science student. Together, the trio has been working to deploy devices called accelerometers as a way to measure chipmunk behavior.
Big Answers, Small Packages
Accelerometers, which record acceleration along multiple axes, are gaining ground in ecology because they facilitate quantitative study of nature’s most clandestine members, from hippos to Griffon vultures—and, now, chipmunks. Of course, these gadgets bring their own suite of problems: optimizing them for use on chipmunks has been no easy feat, and making sense of the data they produce is a research project in and of itself.
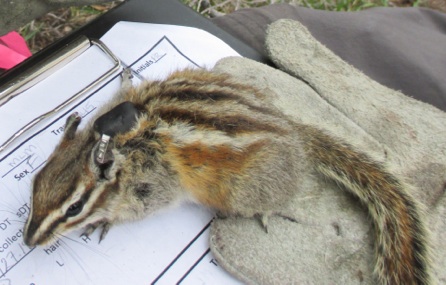
With help from Springthorpe, who modified the accelerometer’s design to suit its tiny wearers, Hammond has piloted methods for building and attaching the devices. She needs the instruments to stay in place on chipmunks for long enough to collect useful data, but also wants them to fall off quickly once their batteries have expired.
“So we decided to try glue,” she says, laughing. After various trials, Hammond says, “We used this cyanoacrylate glue called Blink Lash glue,”—none other than eyelash extension glue—“and it works really well.”
Keeping the accelerometers in place, however, is only half the battle. Before it has been processed, accelerometer output is simply a maze of line plots changing through time. In order to tease information about behavior from this tangle, Hammond partnered with Berg-Kirkpatrick to apply a special statistical method—a system called machine learning—for translating the accelerometer readings into biologically useful data.
(Found) in Translation
Machine learning works because a chipmunk on the run produces a signature accelerometer output that differs in quantifiable ways from a chipmunk that is stationary or moving in place. And the more instances of running that the machine learning system observes, the more accurately it is able to characterize a behavior.
“Machine learning’s not magic,” Berg-Kirkpatrick explains. “If you only have a hundred examples, it could learn some very coarse distinctions. But if you have a hundred million examples, you can learn to translate into French from English.”
Although Hammond had limited chipmunk behavioral examples on which to “train” the machine learning system, she and Berg-Kirkpatrick have been able to predict three categories of behavior—in-place movement, locomotion, and no movement—with over 80 percent accuracy. And while distinguishing finer-grain behaviors such as scratching and nesting would be biologically invaluable, both Hammond and Berg-Kirkpatrick are cautiously pleased with the accelerometers thus far.
Nifty gadgetry aside, the use of accelerometers is a technological breakthrough that may allow Hammond to integrate behavior with her findings on stress physiology in ways that were never before possible for her study system.
A Tale of Two Chipmunks
For example, Hammond has already determined that the alpine chipmunk has a significantly greater physiological stress response to being handled than does the lodgepole chipmunk. Likewise, alpine chipmunks demonstrate significantly higher stress levels in captivity than when they are caught in the field, compared to their lodgepole counterparts.
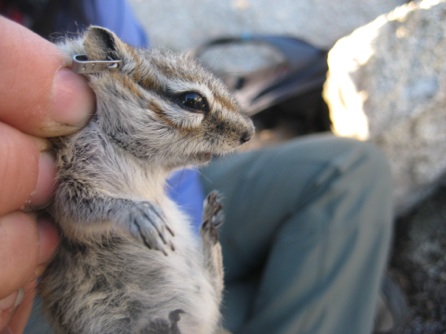
“So there’s some indication—again, it’s all very preliminary—that the alpine chipmunk is more responsive stress-wise than the lodgepole chipmunk,” Hammond says, “And in some way, it is more stress responsive to human-related challenges.” Indeed, some of the sites from which the alpine chipmunk has disappeared are not only at lower elevations, but also are higher human impact sites, often near a road.
Being able to mechanistically answer why the alpine chipmunk’s stress responses vary this way is the crux of Hammond’s drive to obtain good behavioral data, despite the intense labor required to get it.
“My thought is that knowing something about what individuals are doing might reveal some interesting patterns about what times of day the animals are active. So, for example, if the alpine chipmunk is more temperature sensitive, you might expect them to be more active when it’s cooler out.”
And while climate change is her leading hypothesis for now, she says, “I think that other types of human impacts could also be playing a role.”
Hammond presented the results of this research at the 2015 annual meeting of the Society for Integrative and Comparative Biology in West Palm Beach, Florida.
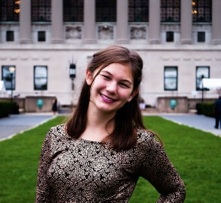
Laura Booth is a recent graduate of Columbia University, where she earned her BA in environmental biology. Her undergraduate thesis research, conducted under Dr. Dustin Rubenstein, used molecular techniques to explore avian malaria in a population of Kenyan starlings. She will join the Golden Gate Raptor Observatory as a research intern studying raptor migration in the summer of 2015. She is also fascinated by science writing and the challenges of making science accessible to diverse audiences.