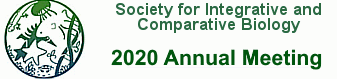
Meeting Abstract
Organisms such as mantis shrimp and trap-jaw ants achieve amazing kinematic output using elastically-driven rather than purely muscle-driven power. In these latch mediated spring actuated (LaMSA) systems, an actuator (muscle) deforms a spring to store energy, with the loaded spring held in place using a latch. When the latch is removed, the stored elastic energy is rapidly released. During this unloading phase, the elastic material undergoes a large deformation at an extremely high rate. Examples of LaMSA systems in nature utilize a variety of biological spring materials and can differ in size by many orders of magnitude. Open questions remain about how materials and size-scale affect the limits of kinematic performance in LaMSA systems. Previous work has focused on the effects of size-scale and the degree of loading on elastic unloading. At constant degree of loading, velocity was independent of size-scale, while acceleration became larger for small systems. At high rates of unloading, materials became less efficient at releasing elastic energy. Our current work continues to explore the effects of and coupling between parameters such as size-scale, degree of loading, materials, and geometries. We focus on connecting the ideas of energy efficiency of elastic materials to more traditional materials testing approaches that can be applied to elastic biomaterials. Using a series of synthetic elastomers, we find a relationship between the resilience measured in a tensile test and the phase angle measured with dynamic mechanical analysis. By probing the fundamental materials physics of composite elastic structures, the biomechanics of latch-mediated spring actuated organisms can be better understood.