My brain works to control only two feet, and I still manage to trip on a regular basis. Sea stars on the other hand, have hundreds of tube feet and no brain, but somehow keep walking. In fact, sea stars are capable of a speedy bouncing gait characterized by vertically oscillatory motion relative to the ground. During this bouncing gait, about a third of their plentiful, hydraulically-powered tube feet must move synchronously (almost as if the sea star is making an escape on 100 tiny pogo-sticks). Theodora Po, a fifth year PhD candidate at the University of California Irvine (UCI) in Dr. Matt McHenry’s lab, has been intrigued by this system and is attempting to answer the question: how does such a simple nervous system accomplish such a complex, coordinated motion?
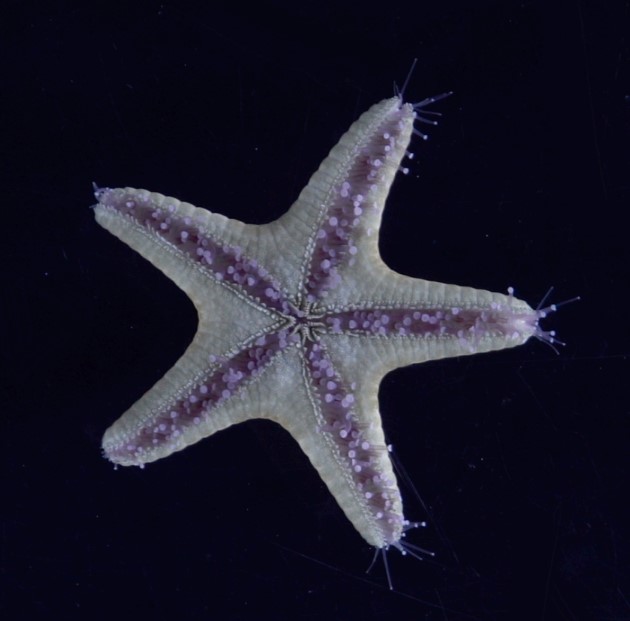
Po hypothesized this was accomplished through mechanical coupling, specifically “Huygens synchronization” in which there is a physical link to separate parts. Familiar examples of this phenomenon include: pendulum clocks hanging from the same beam eventually synchronizing to the same period and amplitude, or when metronomes coupled to the same physical surface all end up moving in unison despite all starting in a random orientation. In both cases, the physical link (the beam of the clocks or physical surface of the metronomes) is moved just a little bit each time by the separate moving parts. Although seemingly negligible, eventually all those separate pushes and pulls sync up to move in unison. Perhaps tube feet function like pendulum clocks and metronomes during the bouncing gait of sea stars! Po took a three-pronged approach to answering this question using a numerical model, experimental manipulation, and a robot.
In the numerical model, Po had each tube foot represented by two springs – one linear and one torsion spring. All the feet started out at a random orientation without centralized control, just like a sea star. The “feet” (separate parts) in the model move along the substrate attached to the “body” of the sea star (the physical link), When feet are engaged at a similar angle at a similar time, this indicates synchrony, and that is exactly what the model demonstrated. So, in theory: YES! Mechanical coupling is sufficient to achieve synchrony in this system without centralized control, just the shared physical ljnk.
Next up was experimental manipulation. Po observed that synchrony only occurred at a certain weight, therefore the next step was to see how the weight of the sea star impacted synchrony of the tube feet. Weights and floats were attached to sea stars (Protoreaster nodosus) all appropriately named “Patrick.” Motivating and determining which “Patrick” was ready and willing to bounce was one of the trickiest parts of these experiments. But once successful, Po identified the impact of differing weights on synchrony by testing a range of values from 50 – 150% submerged body weight. This indicated that increased weights led to an increase in recruitment of tube feet during the powerstroke, so higher weights led to higher synchrony with only a slight decline in bouncing speed.
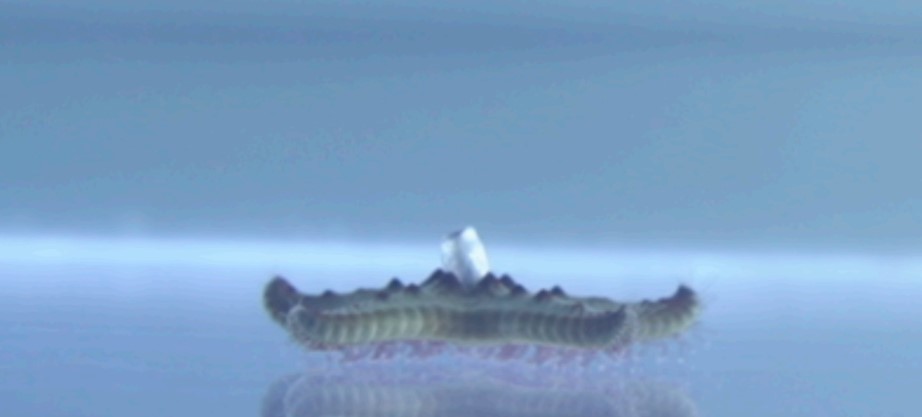
With this idea in mind, Po went back to the numerical model to clarify the phenomenon by looking at the force exerted on the tube feet. The model indicated that submerged weight creates a force threshold that the feet must overcome to move forwards and upwards during the bouncing gait. This means enough feet have to move together to overcome this threshold. In short: increased submerged weight leads to an increased force threshold, which requires more feet moving in unison to overcome.
To truly put this hypothesis to the test, Po built a robot with ten velocity-controlled servos affixed to an acrylic chassis to act as the feet of this sea star robot. All servos started at a random initial orientation and were set to the same velocity. Just like the model and the sea star itself, there was no centralized nervous system telling the “feet” when to come in contact with the ground. What do you think they observed? Once again, with increased weight came an increase of synchrony in the system. The unweighted machine had groups of servos moving in unison, but at 160% weighted, all ten servos were moving in unison! This suggests, once again, that yes, mechanical coupling is sufficient for this collective movement. Just like more tube feet needed to be recruited to overcome the force threshold of the weighted sea stars, more servos needed to work together to do the same. Future investigations will include the incorporation of accelerometers and force sensors to collect velocity measurements and force data.
The implications of this study are far reaching if we consider the broader picture: collective control creates a response to loading. This type of collective behavior is observed far outside the realm of sea star feet. Multi-agent systems such as groups of ants and molecular motors also have to increase the number of agents as the load gets heavier, without centralized nervous control. This could have major implications in the creation of complex robots by decreasing the computational power needed to achieve complex motion. By emphasizing the importance of mechanics in animals with simple nervous control, a lot can be achieved with a little.
Theodora Po was the winner of the Mimi Koehl and Stephen Wainwright Award for Best Student Talk in the Division of Comparative Biology at the SICB 2023 meeting in Austin, TX for this work. Po credits the success of the presentation to an interest in music and poetry that helped to tell the story, as well as the artistic skills of advisor and co-author Matt McHenry. The Center of Organismal Biology Group at UCI also was a clear inspiration for the authors over the years, allowing them to see great presentations and hear feedback on a weekly basis. The grants that supported this project include the Office of Naval Research (ONR) grant number: N00014-17-1-2062 and National Science Foundation (NSF) grant number: IOS-2034043.
Samantha Trail is a PhD student at Florida Atlantic University and advised by Dr. Jeanette Wyneken. She is broadly interested in how and why animals move, investigating those questions through a variety of lenses (behavior, sensory biology, biomechanics, etc.). Her current dissertation research focuses on morphological differences among sea turtle species and how those differences impact locomotion and dispersal at various life stages. When she is not in the lab, she enjoys playing soccer and frolicking on the beach with her pup, Wally.